Research
Click each theme for details.
1. Liquid-liquid phase separation (LLPS) and neurodegenerative diseases
In neurodegenerative diseases such as amyotrophic lateral sclerosis (ALS) and frontotemporal dementia (FTD), protein aggregates are often observed in patients' tissues, but how these aggregates form remains unknown. Recently, it has been reported that these aggregates are formed via a process called liquid-liquid phase separation (LLPS).
LLPS is a phenomenon in which solutes bind to each other multivalently when mixed in an aqueous solution, forming droplets in the solution and separating as if they were water and oil. It has been reported that many ALS-causing proteins undergo LLPS, and furthermore, it has been shown that the phase transition (change of state from liquid to solid) occurs via phase separation.
Poly(PR) and poly(GR) peptides produced from C9ORF72, the most frequent causative gene of familial ALS, have been shown to bind to other molecules due to their strong positive charge and actually, phase-separate with various molecules and exhibit toxicity. However, the molecular mechanism has remained unclear. By combining conventional molecular biology and biochemistry with state-of-the-art quantitative proteomics and data mining techniques, and molecular dynamics simulations, we have elucidated how these dipeptides regulate phase separation and exert toxicity (Kanekura et al. Hum Mol Genet 2016; Kanekura et al., Sci Rep. 2018; Chen et al., J Cell Biol. 2021; Miyagi et al., iScience 2023). We believe that elucidating the pathogenesis of C9ORF72-related ALS will significantly contribute to ALS research.
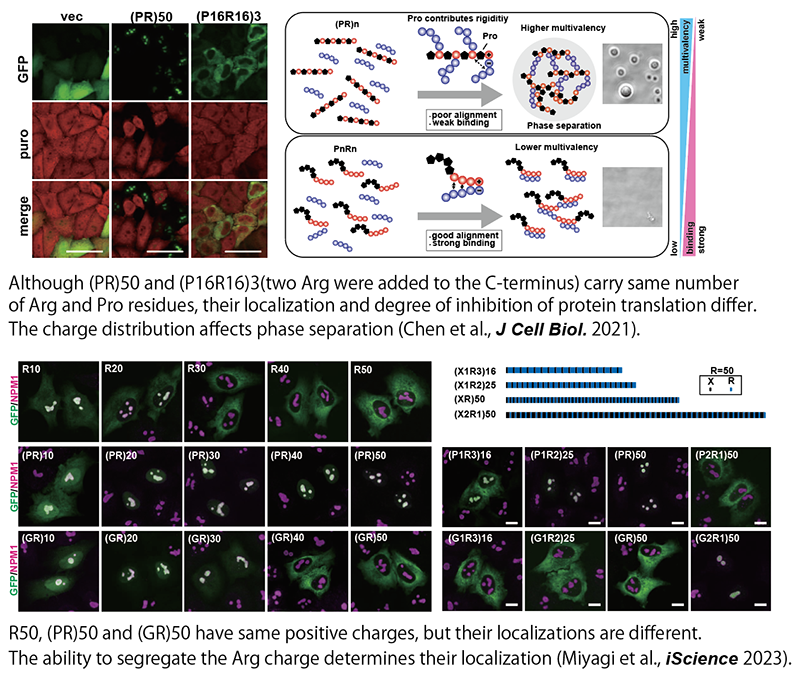
TDP-43 is also known to be an LLPS-prone protein, and it is one of the major components of the inclusion body found in ALS tissues. However, little is known about how it forms aggregates. Optogenetics studies have shown that TDP-43 aggregation is induced by a phase transition via LLPS. We developed a technique to control TDP-43 phase separation using a small compound and confirmed that TDP-43 can be localized from the nucleus to the cytoplasm by the addition of the compound, inducing aggregates (Yamanaka et al. Lab. Invest. 2020; Kanekura et al., Neur. Regen. Res. 2022). Further analysis will reveal the relationship between TDP-43 phase-separation abnormalities and ALS pathogenesis.
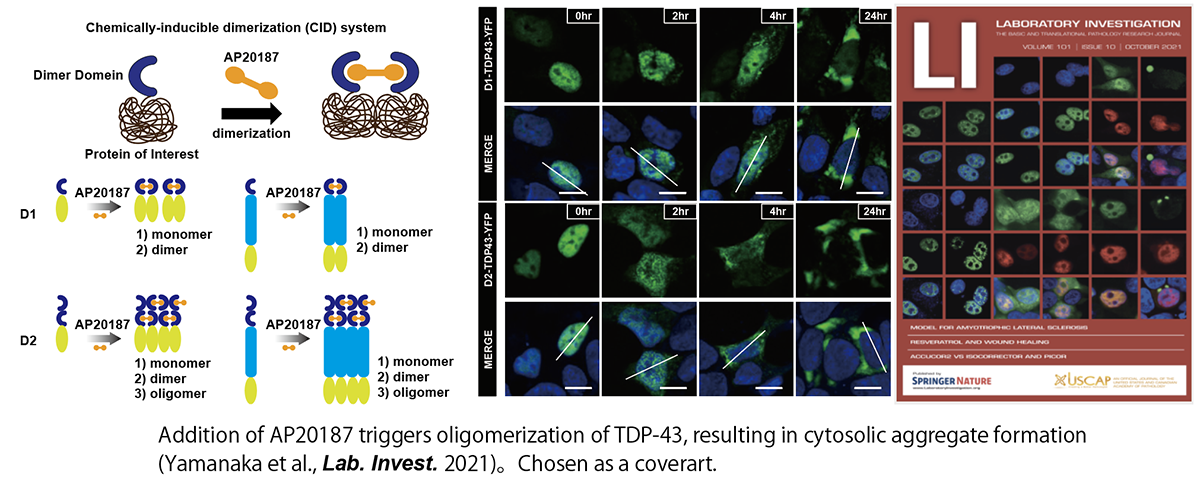
2. LLPS and membrane-less organelles (MLOs)
LLPS is not only involved in the pathogenesis of ALS and other neurodegenerative diseases but is also essential for various physiological functions in cells. In particular, it has attracted particular attention as a mechanism for the formation of membrane-less organelles (MLOs), such as nucleoli, which do not have the lipid-isolated membranes of conventional intracellular organelles. However, the mechanism of MLO formation by phase separation of specific molecules at specific sites in eukaryotic cells, where extremely high concentrations of proteins, nucleic acids, and other macromolecules are present, is still largely unknown. We focused on sequences specific to a group of proteins constituting MLOs called nuclear speckles. It is known that many of the proteins in the nuclear speckle have sequences in which the acidic amino acids glutamic acid (D) and aspartic acid (E) alternate with the basic amino acid arginine (R) (Greig et al., Mol Cell 2019). We generated a large number of mutants of these sequences and analyzed their subcellular localization and phase separation, and found that the MLOs localized differently depending on the arrangement of the sequences, even if the sequences contained the same number of Ds and Rs. This indicates that the alternating presence of D and R is essential for localization to the nuclear speckles (Miyagi et al., Int J Mol Sci. 2022). Data mining of the human proteome revealed that alternating sequences of R and other amino acids act as endogenous MLO-targeting signals that regulate localization to the nucleolus, nuclear speckle, and PML body (Miyagi et al., iScience 2023). In the future, we will further elucidate the mechanism of localization to specific MLOs and the mechanism of MLO formation.
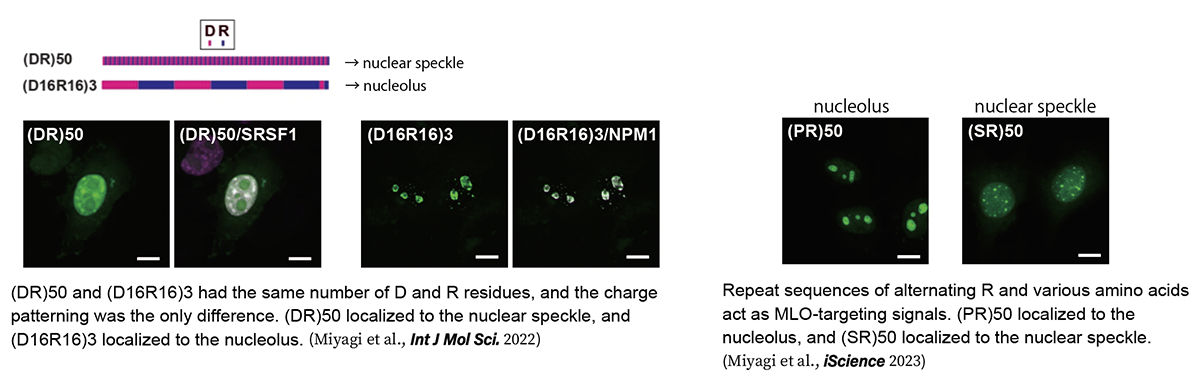
3. Visualization of Macromolecular Crowding level in phase-separated droplets
High molecular crowding is critical for macromolecules to undergo LLPS. In 2015, a crowding sensor based on FRET was reported (Boersma et al., Nat. Method. 2015), and changes in intracellular crowding under various stresses were measured. However, the sensitivity as a sensor was not sufficient, and it was difficult to detect minute changes. Therefore, we developed CRONOS (crowding sensor with mNeonGreen and mScarlet-I), a sensor with higher sensitivity than conventional sensors, by optimizing the pair of donor and acceptor fluorescent proteins of FRET. which enables the detection of crowding changes inside MLOs under stress, a previously unknown phenomenon (Miyagi et al., BBRC 2021), and the first visualization of environmental changes inside phase-separated droplets (Miyagi et al., iScience 2023). In the future, we would like to apply this technique to macromolecular crowding measurements in vivo and clarify how the degree of macromolecular crowding is controlled and changes in vivo. By continuing this research, we will be able to clarify questions such as why proteins aggregate in certain cells or exhibit toxicity in certain cells.
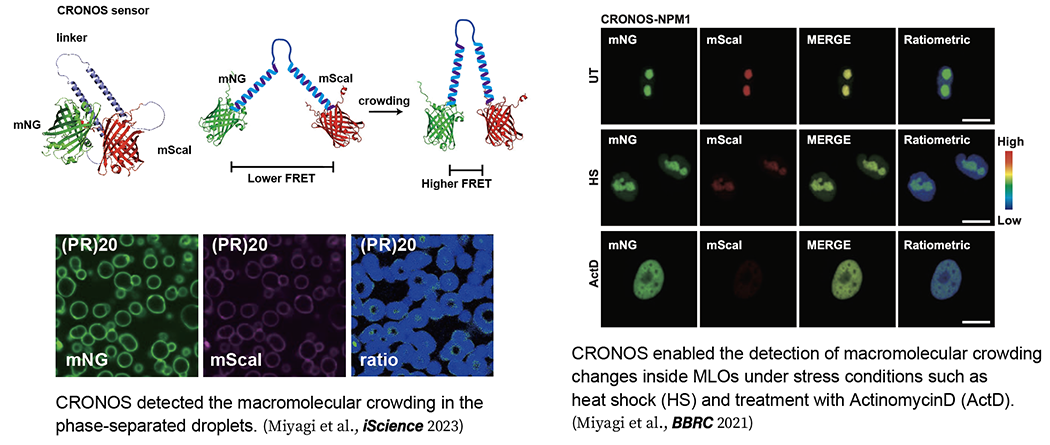
4. Endoplasmic Reticulum Stress and Neurodegenerative Diseases
Unlike familial ALS, in which genetic mutations are detected, the pathogenesis of sporadic ALS, in which there is no family history, is largely unknown. However, since it has been shown that intracellular stress called endoplasmic reticulum (ER) stress is induced in the neural tissue of patients with sporadic ALS (Saxena et al., Nat Neurosci. 2009), we have been studying the relationship between ER stress and neuronal cell death. The ER is an intracellular organelle that synthesize membranous and secretory proteins and also plays important roles in the addition of glycans to these proteins and in intracellular calcium storage. It is also the site of disulfide bond (-S-S-) formation, which confers structural rigidity to the membranous proteins and secretory proteins, and thus maintains exceptionally oxidative conditions inside. We developed a probe, MERO-GFP (mammalian ER redox-GFP), to visualize the redox potential in the ER and observed changes in the ER environment under ER stress (Kanekura et al., Lab. Invest. 2012). We discovered a novel mechanism by which the redox potential is disturbed by increased permeability of the ER membrane under ER stress, resulting in the induction of cell death (Kanekura et al., Mol. Endocrin. 2015; Kanekura et al., Science Signaling 2015).
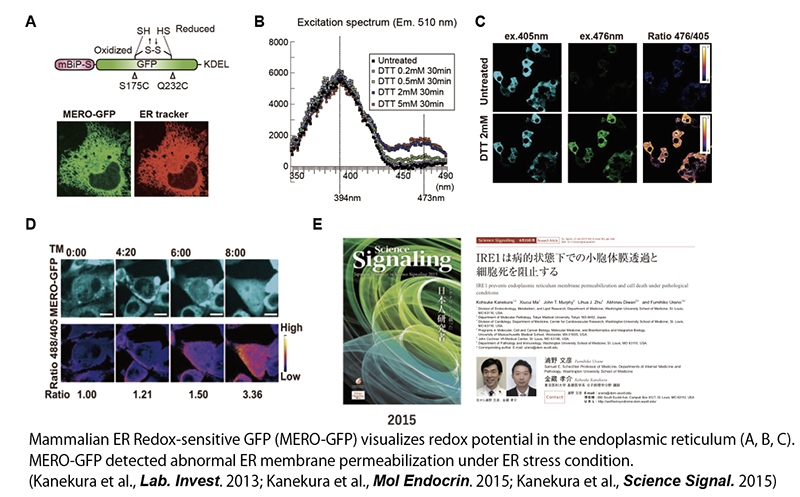
5. Elucidating disease pathogenesis mechanisms using multi-omics analyses
We are also collaborating with physician-scientists from various clinical departments. We identified PLK1, a gene essential for triple-negative breast cancer (TNBC) growth, by siRNA screening in collaboration with the Department of Breast surgery, Tokyo Medical University, and reported on the potential use of its inhibitor for TNBC treatment (Ueda et al. Lab. Invest. 2019). In collaboration with the Department of Hematology at Tokyo Medical University, we also identified the gene LAT1, which is essential for the growth of malignant lymphomas, and reported that its inhibitor could be a potential therapeutic candidate for malignant lymphomas (Jigjidkhorloo et al., Sci. Rep. 2021). Furthermore, in collaboration with the Department of Ophthalmology at Tokyo Dental University, we have revealed the importance of the environment in the aqueous humor as a cause of iris atrophy by multi-omics analysis (Yamaguchi et al., Science Advances 2020). We will continue to collaborate with clinical faculty members.
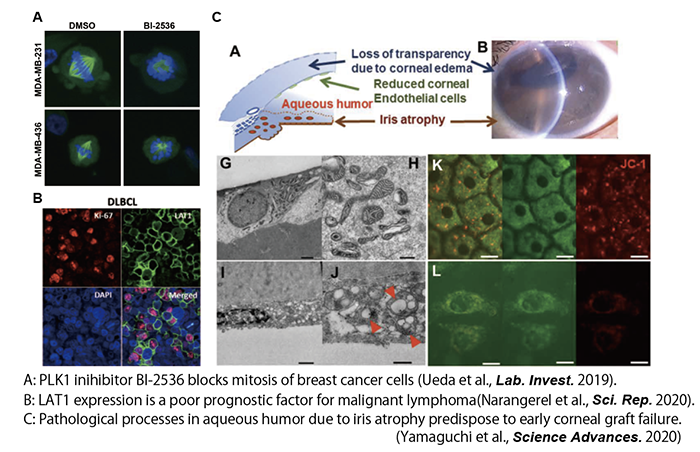